
The Glow
It’s dark. You can’t see much, but suddenly tiny flashes of yellow-green lights illuminate the night.
A swarm of fireflies.
For centuries, these strange little bugs have captured imaginations with their ability to produce their own light. Other creatures, including jellyfish, can do it too. It’s a phenomenon of nature called bioluminescence, and the glowing proteins responsible for it are revolutionizing the world of biotechnology.
“We don’t yet understand all the facets of bioluminescence, but scientists have harnessed its glow and are using glowing proteins for everything from tracking the process of bacterial infections to detecting chemical and biological agents planted by terrorists,” says Marc Zimmer, the Jean C. Tempel ’65 Professor of Chemistry.
Zimmer is a computational chemist who works with green fluorescent proteins (GFP). A few doors down in Hale Laboratory, Bruce Branchini, the Hans and Ella McCollum ’21 Vahlteich Professor of Chemistry, runs one of only three labs in the country researching the bioluminescent pathways of fireflies.
By using glowing proteins as tracers in cancer to bacteria to stem cells, scientists can see proteins being made and moving in real time. Proteins are extremely small and can’t be seen even with an electron microscope. But when they give off light the brightness can be detected.
Zimmer uses this analogy: “Imagine you are standing at one end of a football field at dusk. There’s no way you’d be able to see a little bug all the way across the field. But then that bug—a firefly—lights up, and suddenly, you can see it.
“It’s like the microscope. It’s the modern way of seeing.”
Nobel Prize for GFP
Doctors have noticed a strange trend. Pregnant women who have had heart attacks seem to recover better than other women and men.
“The doctors developed a theory: When someone has a heart attack, a distress signal is sent for other cells to come help, but none respond,” says Zimmer. “But if the woman is pregnant, embryonic stem cells go through the bloodstream to help mend the heart.”
But how to prove it?
Hina Chaudhry, an associate professor of medicine at Mount Sinai School of Medicine, had an idea. Researchers in her lab took a normal female mouse and bred it with a male mouse genetically modified with GFP to produce glowing offspring. After the female gave birth, no fluorescent proteins remained in her body. But when they repeated the experiment and induced a heart attack, glowing cells were visible in the injured area of the pregnant mouse’s heart.
“Using GFP, Chaudhry was able to prove that the stem cells of the fetus quite literally mended the mother’s broken heart,” Zimmer says.
It’s one of Zimmer’s favorite examples of GFP use in medical research, but there are countless others. GFP, which was first cloned in 1994, is now used for hundreds of thousands of experiments each year by scientists across the world.
Justine Miller ’07 is one of them. A senior scientist at the biopharmaceutical company AbbVie, Miller uses GFP in her research into neurological disorders.
“There are certain proteins that are key hallmarks of diseases like Alzheimer’s—proteins that pass from one cell to another. If we tag them with GFP, we can track what is happening to them inside of a live cell culture,” she says.
While GFP is now a staple in scientific labs (it’s so common that GFP-modified fish are now sold in pet stores), it was a relatively unknown molecule when Zimmer first learned about it during a campus lecture in 1995. Intrigued by the possibilities for GFP, he wondered if he could use computer models to determine the underlying chemistry that made it fluoresce. He has since focused his research efforts on trying to better understand what makes certain proteins light up, how to manipulate them to glow brighter, and how to more easily change their colors and turn them on and off.
With funding from the National Institutes of Health and the Research Corporation for Scientific Advancement, Zimmer has published dozens of papers in academic journals, including many co-authored by student researchers. But perhaps his greatest contribution to the field is the work he has done to tell just about everyone about it. He is the author of Glowing Genes, the first popular book about jellyfish proteins and firefly bioluminescence, and Illuminating Disease, which details GFP’s role in biomedical research from malaria to HIV. He also runs a GFP website that has become popular among high school science teachers.
In 2008, the Royal Swedish Academy of Sciences—the organization responsible for awarding the Nobel Prize—invited Zimmer to Stockholm to answer questions about GFP and the scientists who pioneered its study. Later that year, the Academy awarded the Nobel Prize in Chemistry to three of those scientists; the official announcement included a link to Zimmer’s website.
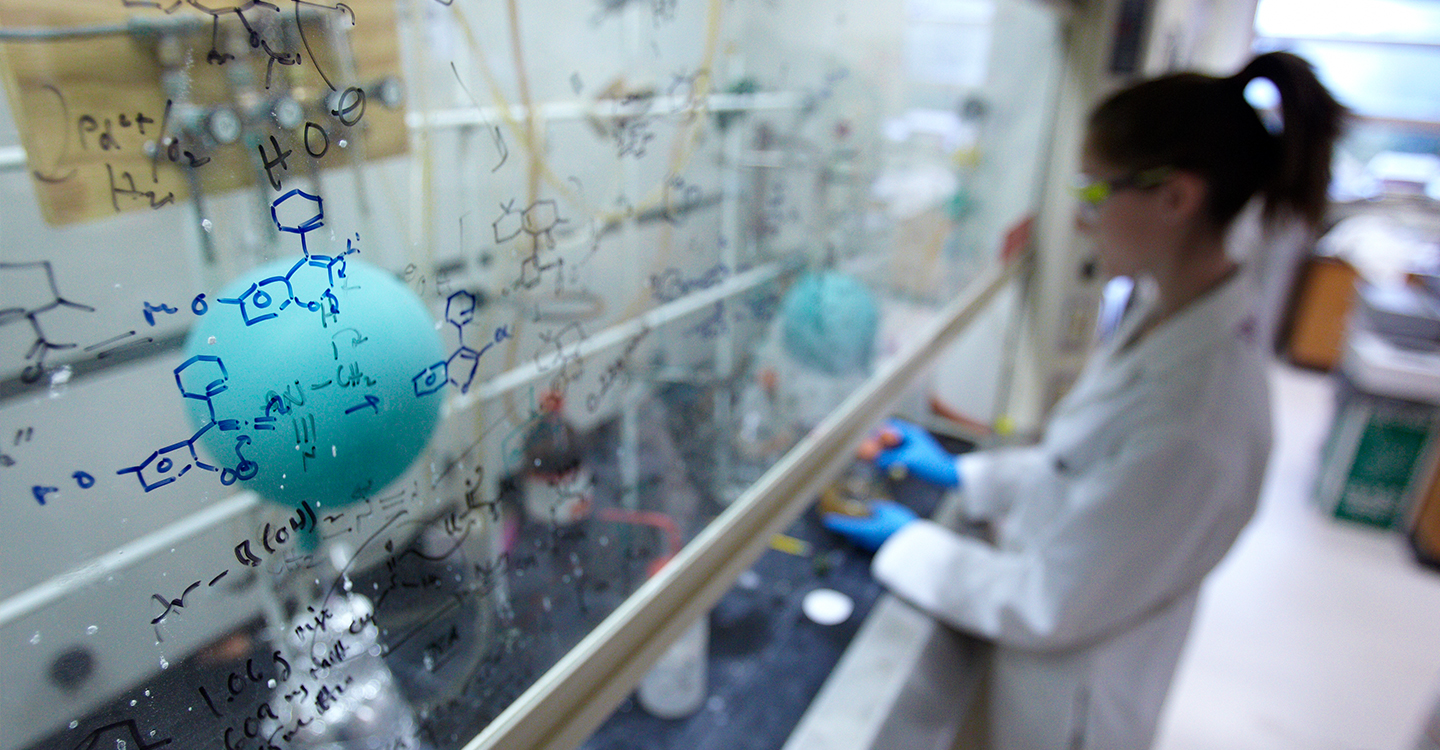
Lightning Bugs
A yellowed 1987 newspaper clipping hangs on the door of Branchini’s laboratory. In the article, the professor says he hopes to someday solve the mystery of exactly how the firefly glows.
Two years ago, he did just that.
To produce light, you need energy, Branchini explains. Fluorescent proteins like GFP require an external light, like a black light, to glow. The bioluminescence of the firefly doesn’t require any external light; instead, a chemical reaction takes place inside the bug’s body.
For decades, the widely held theory among scientists was that the reaction happened when regular oxygen mixed inside the firefly’s abdomen with calcium, adenosine triphosphate and the chemical luciferin in the presence of the enzyme luciferase. But Branchini had another theory; he believed the reaction was actually set off by a highly reactive form of oxygen called superoxide. After decades of work with firefly luciferase, he was convinced. But because of the speed of the reaction, he wasn’t able to produce definitive proof.
Then he got an idea, and it involved a short trip down I-95 to Yale University. There, researchers would use a technique called electron paramagnetic resonance (EPR) to try and make the superoxide stable enough to measure.
It worked perfectly on the very first try. Branchini was able to measure the superoxide and definitively prove it was this form of oxygen that makes these lightning bugs glow.
“We were right. Even the postdoc who ran the EPR couldn’t believe it. There it was—the evidence we needed. The final piece of the puzzle,” Branchini says.
It was a crowning moment in the career of one of the leading researchers in firefly bioluminescence in the world.
Branchini’s research group—which includes three technicians and, at any given time, several undergraduate students—has published more than 50 scholarly articles in the last 13 years alone. Nearly all of Branchini’s work is funded with grants, mainly from the National Science Foundation and the Air Force Office of Scientific Research, which has awarded Branchini nearly $1.7 million to date.
“The Air Force is interested in using bioluminescence as a marker. They want us to transform bioluminescence you can see into an area of light you can only see with something like night vision goggles,” Branchini says.
Branchini isn’t privy to exactly how the Air Force applies his work, but it could be used to create a helicopter landing pad invisible to the naked eye and undetectable by heat sensors. The underlying technology, however, could also have implications far beyond national defense.
“The near infrared technology that the Air Force is interested in is also important for the bioimaging of live animals, because near infrared travels best through tissues,” he says.
By working to better understand the biochemistry of bioluminescence at its most basic level, Branchini and his team can produce luciferase proteins with different properties ideal for different applications, from drug screening to mechanistic studies to in vivo imaging, a noninvasive technique used to track molecules inside of living animals.
Conn holds a U.S. patent (and the corresponding European patent) for one of these proteins, an enhanced version of an enzyme from an Italian firefly. After cloning the gene for the enzyme and determining its DNA sequence, Branchini and his team were able to stabilize it and change its color from yellow-green to an orange-red. The patent is currently licensed to Targeting Systems, Inc., a California-based company that offers unique luciferases for use in drug development and other research.
Another red-glowing enzyme developed by the team has proven particularly popular with infectious disease researchers.
“We get hundreds of emails requesting it,” Branchini says. “It’s difficult to get funding to study some rare diseases, so it’s nice to know our work is doing some good in the world.”
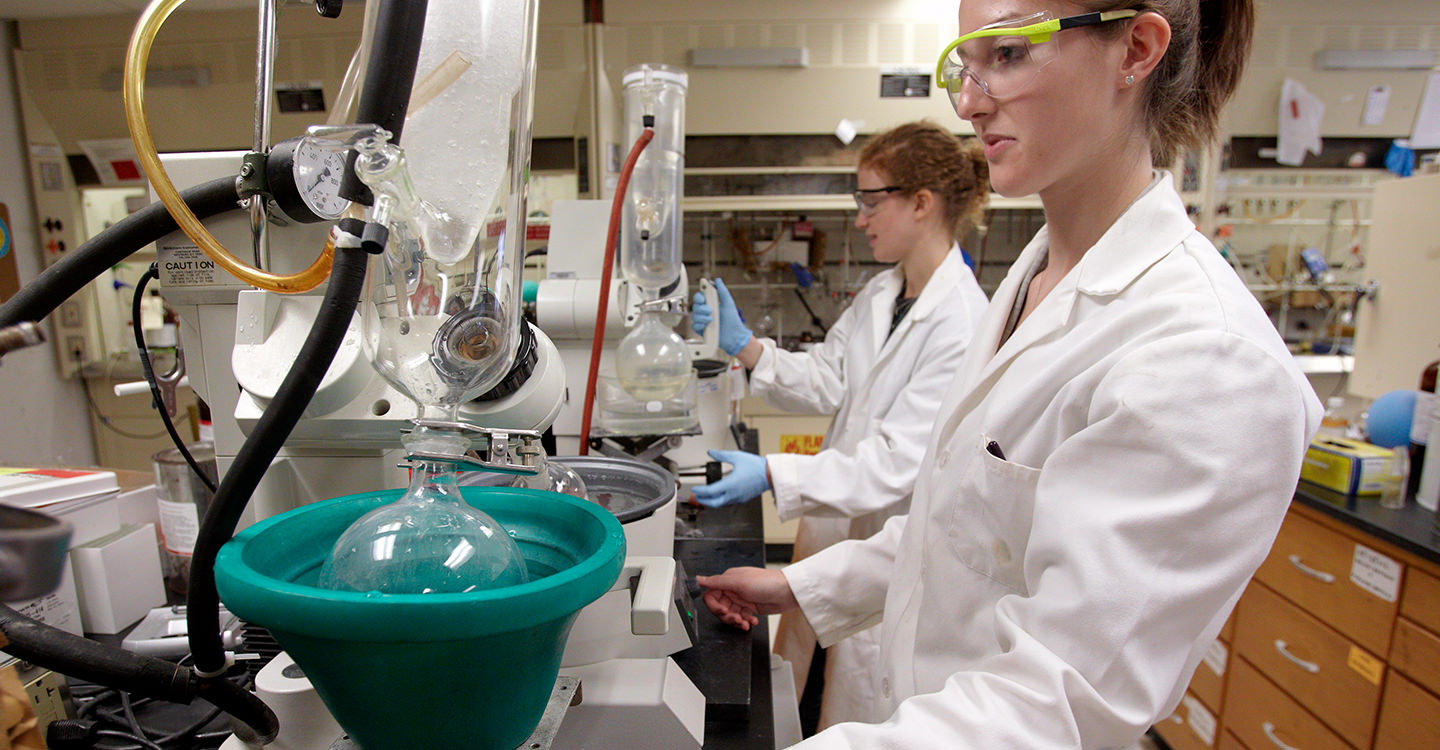
A New DNA
The entire genetic alphabet—the building blocks of all life on earth—is made up of just four letters: A (adenine), T (thymine), C (cytosine) and G (guanine). And since A is always paired with T, and C with G, these letters make up the two DNA base pairs that are used to make all proteins in all living cells on earth.
Until now.
At the Scripps Research Institute in California, chemist Floyd Romesberg and his team, including Aaron Feldman ’13, have added two more letters—a third base pair.
“The idea is with a six-letter alphabet, we can make more ‘words’ and ‘sentences’—amino acids and proteins,” says Feldman, co-author of the groundbreaking paper published in the November issue of Nature.
Other researchers have added unnatural base pairs to DNA before, but they’ve never been used to actually make a new protein. To prove it is possible, Romesberg, Feldman and their colleagues created a strain of E. coli bacteria that used the new base pair to produce a genetically modified version of GFP.
Sure enough, it glowed.
“This is the first time ever a cell has translated a protein using something other than G, C, A or T,” Romesberg said in a release announcing the study. “I would not call this a new life-form—but it’s the closest thing anyone has ever made.”
The four natural DNA bases are used by organisms to produce 20 different amino acids. With the additional two letters, organisms could produce up to 152 new amino acids, which could be used to make new, more efficient medicines.
“GFP has been the workhorse in this type of research for a long time,” says Feldman. “It’s incredibly useful and abundant. Everyone who can take advantage of it does.”
That won’t change anytime soon, Zimmer says. In fact, he predicts all of today’s students who pursue careers in biology or medicine will use fluorescent proteins.
And the possibilities are nearly endless. Fluorescent proteins could be used to help remove cancerous cells in humans, for example.
“There’s bacteria that collects on the margins of tumors. You can make that bacteria fluoresce. One day, doctors may be able to turn on a blue excitatory light during surgery and see additional tumors they couldn’t see before,” says Zimmer.
The ability to image deep within the brain is also a conceivable possibility in the future. And the burgeoning field of optogenetics, in which light is used to control neurons in the brain, is another example of the incredible power of the glow.
“Glowing gene technology is really just in its teenage years,” says Zimmer. “We’ve only begun to unlock its potential.”